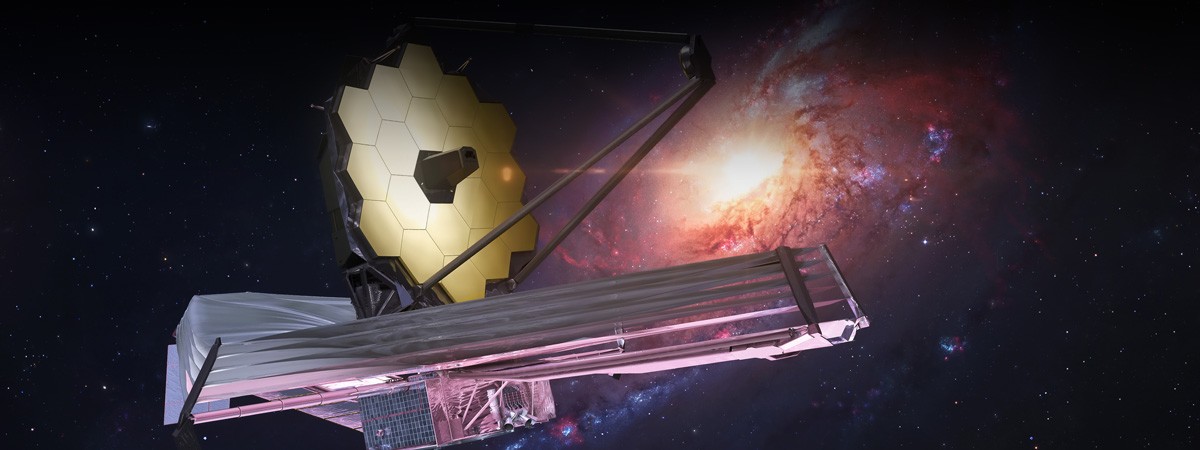
A Sharp Glance Into The Distant Universe
The smoothest mirrors for the brightest images
“Bumpy” is not a word you would usually associate with the smooth glass of a mirror. But compared to the mirrors inside the giant optical telescopes found in observatories, the typical household mirror is covered in imperfections. To achieve the perfect mirror needed for accurate insights into distant galaxies, the reflective surface is leveled by an ion beam under vacuum, using vacuum pumps from the Busch Group.
Inside an observatory, giant concave mirrors are the main characters. With careful positioning, they focus the light that hits them from the depths of space, allowing researchers to see way beyond the reaches of our galaxy without ever leaving the Earth’s surface. To give a clear picture, these mirrors must be smooth to a tolerance of just tens of nanometers – eliminating unevenness as impossibly small as a single strand of DNA. This level of precision is only possible under vacuum in a process called ion-beam figuring.
Scattered light and reflective surfaces
Every material reflects light to some degree. Think of all the places you’ve spotted your reflection – a car door, a puddle, a polished floor. All of these have one thing in common: They are smooth. Rough surfaces, even those with quite high light reflectivity like white concrete, scatter the light that hits them. This allows us to see the surface itself, but no reflection. Although the difference is too small for our eyes to detect, this is one reason why our mirrors at home would be unsuitable inside a precision telescope. They only reflect around 90% of the light that hits them: more than enough to check your outfit before leaving the house, but not enough to accurately reflect the faint light from the other end of the universe. For this, we need special processing to ensure the smoothest possible surface and highest reflectivity.
Maintaining focus
Ion beam figuring is the final smoothing stage in creating a mirror. Beforehand, the glass has been ground and polished to an extremely high standard – a process so thorough that it can take months. The now already very smooth glass is placed inside a vacuum chamber, where a vacuum pump removes the ambient air. An ion beam, a focused stream of positively charged argon ions, then traces over the surface of the mirror, line by line. This ion beam hits any molecules that are sticking out, physically knocking them out of place and allowing errors on the surface to be removed with accuracy to the nanometer. It is essential that this process takes place under vacuum. In ambient air, the ions can hit air molecules before they reach the glass and are deflected instead. The beam loses energy and the ions scatter. Under vacuum, the beam remains focused. This allows for the precise figuring necessary to create the smoothest mirror – so that we can detect the light of the distant stars here on Earth.
Scattered light and reflective surfaces
Every material reflects light to some degree. Think of all the places you’ve spotted your reflection – a car door, a puddle, a polished floor. All of these have one thing in common: They are smooth. Rough surfaces, even those with quite high light reflectivity like white concrete, scatter the light that hits them. This allows us to see the surface itself, but no reflection. Although the difference is too small for our eyes to detect, this is one reason why our mirrors at home would be unsuitable inside a precision telescope. They only reflect around 90% of the light that hits them: more than enough to check your outfit before leaving the house, but not enough to accurately reflect the faint light from the other end of the universe. For this, we need special processing to ensure the smoothest possible surface and highest reflectivity.
Maintaining focus
Ion beam figuring is the final smoothing stage in creating a mirror. Beforehand, the glass has been ground and polished to an extremely high standard – a process so thorough that it can take months. The now already very smooth glass is placed inside a vacuum chamber, where a vacuum pump removes the ambient air. An ion beam, a focused stream of positively charged argon ions, then traces over the surface of the mirror, line by line. This ion beam hits any molecules that are sticking out, physically knocking them out of place and allowing errors on the surface to be removed with accuracy to the nanometer. It is essential that this process takes place under vacuum. In ambient air, the ions can hit air molecules before they reach the glass and are deflected instead. The beam loses energy and the ions scatter. Under vacuum, the beam remains focused. This allows for the precise figuring necessary to create the smoothest mirror – so that we can detect the light of the distant stars here on Earth.
Read more – Light that the eye cannot see
Even the highly magnified view that we get from a visible-light telescope only shows a tiny proportion of the picture in front of us. The Extremely Large Telescope, the world’s largest optical telescope that is currently under construction in the Atacama Desert in Chile, will gather 100 million times more light than the human eye can perceive. But there is so much more information coming to us from space that our eyes just cannot see.
The wavelength of visible light ranges between around 380 to 750 nanometers. As a comparison, a single human hair is around 90,000 nanometers in width. Radio telescopes help us see what we cannot with the naked eye: Faint and distant stars, otherwise invisible black holes, or the emissions from a gas planet. Every object in the universe emits radio waves – wavelengths longer than the visible spectrum. By examining these, astronomers can gain an even fuller picture of the universe that surrounds us.
Even the highly magnified view that we get from a visible-light telescope only shows a tiny proportion of the picture in front of us. The Extremely Large Telescope, the world’s largest optical telescope that is currently under construction in the Atacama Desert in Chile, will gather 100 million times more light than the human eye can perceive. But there is so much more information coming to us from space that our eyes just cannot see.
The wavelength of visible light ranges between around 380 to 750 nanometers. As a comparison, a single human hair is around 90,000 nanometers in width. Radio telescopes help us see what we cannot with the naked eye: Faint and distant stars, otherwise invisible black holes, or the emissions from a gas planet. Every object in the universe emits radio waves – wavelengths longer than the visible spectrum. By examining these, astronomers can gain an even fuller picture of the universe that surrounds us.