Supercooled superconductors enable current to flow without resistance. Energy loss during transport is minimized. Vacuum helps generate and maintain the required cooling.
Cold eliminates resistance
"Where electricity flows, there is resistance": Dutch physicist Heike Kamerlingh Onnes turned this postulate of electrical science on its head in 1911 when he discovered the phenomenon of superconductivity. Because according to the rules of quantum physics, some materials lose their electrical resistance if they are cooled to very low temperatures. Electricity can thus flow without energy loss. The transport loss in European power grids is approximately six percent. Resistance-free electricity transport could therefore reduce the number of power plants required. However, real-world superconducting cables are a quite complex high-tech construction.
Vacuum protects from heat
Superconductor cables have little in common with conventional power lines: they consist of several layers, like a garden hose with different diameters placed within one another. The live layers contain superconducting strips made from a ceramic material. The transition temperature of such materials – or the point at which the material takes on superconductor properties – is between minus 130 and minus 180 degrees Celsius. These temperatures are considered high in the context of superconductor physics; ceramics are designated as high-temperature superconductors.
But compared to the ambient temperature, extreme cold is still needed. This is why the cable bundle is cooled with cryogenically cooled liquid nitrogen. It constantly flows through the hollow space that surrounds the superconducting layer. The cable also has a coating to guard against external temperature influences with two walls with a vacuum in between – insulation according to the same principle as a thermos flask.
In the AmpaCity pilot project, one such cable runs clear through a kilometre of Essen's city centre between two transformer stations. It passed the practical test during a two-year trial phase. In addition to evacuating the insulating vacuum jacket, this project also uses vacuum technology for cooling the nitrogen. In the cooling unit, vacuum pumps are used to keep the gas at a temperature significantly lower than its boiling point of minus 196 degrees Celsius.
Efficiency gains and medical technology
Despite their complex layered construction, superconductors are significantly thinner than comparable copper cables: they have the same diameter but can transport five times more electricity. They also withstand much stronger current than conventional cables. This is why experts are convinced that the new cable technology will not only be a more energy-efficient solution for transporting electricity in mains supplies, it will also save more space – an essential aspect for the urban project in Essen. Superconductor cables could take over electricity transport from the power lines outside the city borders and dispense with some of the substations.
Superconductors could also make transformers, generators and motors more efficient. If they replace the copper cables, smaller and lighter models will be possible. Light-weight yet powerful electric motors would even be interesting for engines on aeroplanes. Some aircraft manufacturers are already considering such concepts.
Incidentally, superconductors have long proven themselves in research facilities and medical technology. For example, particle accelerators are equipped with superconducting magnetic coils. These types of magnets are also used in medical diagnostics. Thanks to superconductivity, Magnetic Resonance Imaging (MRI) generates extremely powerful magnetic fields that "illuminate" the body without radiation exposure. Vacuum technology also helps with cooling and insulation in such applications.
Busch provides vacuum systems for cooling and insulating superconducting cables and magnetic coils worldwide.
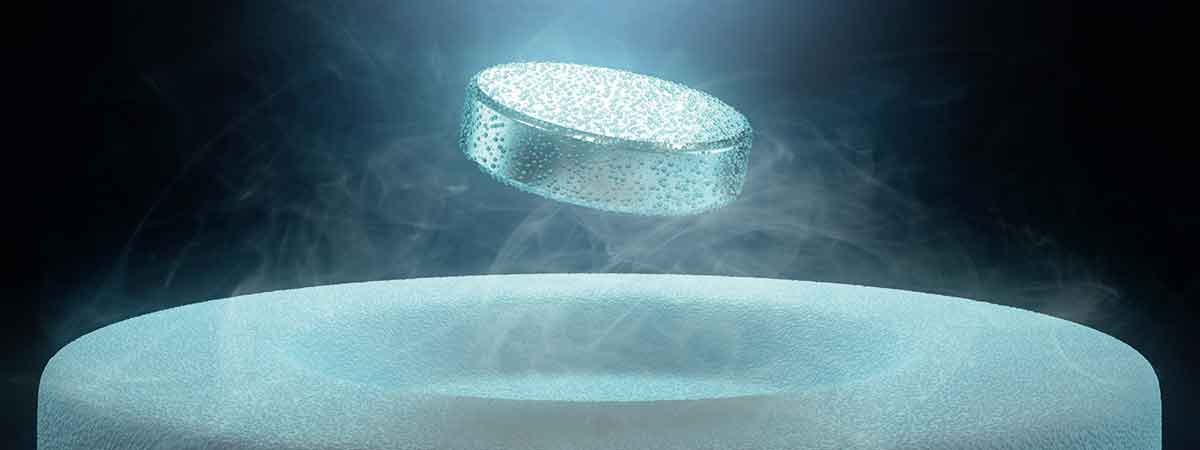
Superconductors for the energy of the future
High-tech cables stay cool thanks to vacuum
Why do we need superconductivity to examine soft tissue?
Highly precise diagnostics with MRI
Magnetic Resonance Imaging (MRI, also sometimes called nuclear magnetic resonance imaging) has become an integral part of medical diagnostics. It provides images of the body with many details that cannot be seen on an X-ray image. With an MRI, a trained eye can also identify injuries to muscles, ligaments, tendons, blood vessels and even nerves. This gives doctors crucial information for surgical procedures or for treating tumour diseases.
The images are produced using extremely strong magnetic fields and radio waves, which cause the hydrogen nuclei in the body tissues to dance. This movement, the nuclear spin, creates electromagnetic waves. These are recorded by the MRI machine and translated into high-contrast images of the body's structures using data analysis. In contrast to X-rays, which are primarily able to display bones, images from MRI machines also show the soft tissues of the body with high detail resolution. Because the machine performs the measurement in millimetre-thin layers, these images can also be compiled to create 3D images. The magnetic field required for the imaging process is 20,000 to 100,000 times stronger than that of the earth, depending on the application. These types of field intensities can only be created and maintained with superconducting magnetic coils.
Highly precise diagnostics with MRI
Magnetic Resonance Imaging (MRI, also sometimes called nuclear magnetic resonance imaging) has become an integral part of medical diagnostics. It provides images of the body with many details that cannot be seen on an X-ray image. With an MRI, a trained eye can also identify injuries to muscles, ligaments, tendons, blood vessels and even nerves. This gives doctors crucial information for surgical procedures or for treating tumour diseases.
The images are produced using extremely strong magnetic fields and radio waves, which cause the hydrogen nuclei in the body tissues to dance. This movement, the nuclear spin, creates electromagnetic waves. These are recorded by the MRI machine and translated into high-contrast images of the body's structures using data analysis. In contrast to X-rays, which are primarily able to display bones, images from MRI machines also show the soft tissues of the body with high detail resolution. Because the machine performs the measurement in millimetre-thin layers, these images can also be compiled to create 3D images. The magnetic field required for the imaging process is 20,000 to 100,000 times stronger than that of the earth, depending on the application. These types of field intensities can only be created and maintained with superconducting magnetic coils.